Unlocking the Strange Secrets of Ice Nucleation
A team of researchers has developed a theoretical model explaining how specific structural details on surfaces influence water’s freezing point, paving the way for advancements in materials design for ice formation and enhanced weather prediction. Credit: SciTechDaily.com
Research unveils a mathematical model for ice nucleation, showing how surface angles affect water’s freezing point, with applications in snowmaking and cloud seeding.
From abstract-looking cloud formations to roars of snow machines on ski slopes, the transformation of liquid water into solid ice touches many facets of life. Water’s freezing point is generally accepted to be 32 degrees Fahrenheit. But that is due to ice nucleation — impurities in everyday water raise its freezing point to this temperature. Now, researchers unveil a theoretical model that shows how specific structural details on surfaces can influence water’s freezing point.
Research Findings and Their Implications
The researchers presented their results at the spring meeting of the American Chemical Society (ACS). ACS Spring 2024 is a hybrid meeting being held virtually and in person March 17-21; it features nearly 12,000 presentations on a range of science topics.
“Ice nucleation is one of the most common phenomena in the atmosphere,” says Valeria Molinero, a professor of physical and materials chemistry. “In the 1950s and 1960s, there was a surge of interest in ice nucleation to control weather through cloud seeding and for other military goals. Some studies addressed how small shapes promote ice nucleation, but the theory was undeveloped, and no one has done anything quantitative.”
Freezing water might seem simple, but not to Yuqing Qiu and Valeria Molinero. By exploring the relationship between surface chemistry and geometry, the process of creating ice or snow could be made more energy efficient, helping create clouds or add snow to mountains. Yuqing will present the research on Wednesday, March 20 at ACS Spring 2024 in New Orleans.
When temperatures drop, the molecules in liquid water, which normally speed around and zip past one another, lose energy and slow down. Once they lose enough energy, they grind to a halt, orient themselves to avoid repulsions and maximize attractions, and vibrate in place, forming the crystalline network of water molecules we call ice. When liquid water is completely pure, ice may not form until the temperature gets down to a frigid –51 degrees Fahrenheit; this is called supercooling. But when even the tiniest impurities — soot, bacteria, or even particular proteins — are present in water, ice crystals can form more easily on the surfaces, resulting in ice formation at temperatures warmer than –51 degrees Fahrenheit.
Advancements in Ice Nucleation Study
Decades of research have revealed trends in how the shapes and structures of different surfaces affect water’s freezing point. In an earlier study on ice-nucleating proteins within bacteria, Molinero and her team found that the distances between the groups of proteins could impact the temperature at which ice formed. “There were distances that were very favorable for ice formation, and distances that were completely opposite,” says Molinero.
Similar trends had been observed for other surfaces, but no mathematical explanation had been found. “People before already had a sense of ‘oh maybe a surface will inhibit or promote ice nucleation,’ but no way to explain or predict what they observed experimentally,” says Yuqing Qiu, a postdoc, who is presenting the work at the meeting. Both Qiu and Molinero carried out this research at the University of Utah, though Qiu now works at the University of Chicago.
To address this gap, Molinero, Qiu, and team gathered hundreds of previously reported measurements on how the angles between microscopic bumps on a surface affected water’s freezing temperature. They then tested theoretical models against the data. They used the models to consider factors that would encourage ice crystal formation, such as how strongly water binds to the surfaces and angles between structural features.
In the end, they identified a mathematical expression that shows that certain angles between surface features makes it easier for water molecules to gather and crystallize at relatively warmer temperatures. They say their model can help design materials with surfaces that would make ice form more efficiently with minimal energy input. Examples include snow or ice makers, or surfaces that are suitable for cloud seeding, which is used by several Western states to increase rainfall. It could also help better explain how tiny mineral particles in the atmosphere help make clouds through ice nucleation, potentially making weather models more effective.
Future Directions in Ice Nucleation Research
The researchers plan to use this model to return to their studies of ice-nucleating proteins in bacteria. More than 200 proteins are believed to be ice-nucleating proteins, but their structures are not all known. The researchers hope to study proteins with structures that have been solved with AI tools, and then they will model how aggregates of those proteins affect ice formation.
Title
The most potent snow makers
Abstract
Several organisms have evolved proteins that control the formation of ice. Ice nucleating bacteria are the most potent ice-nucleating agents in the biosphere and the atmosphere, contributing to cloud glaciation and precipitation, and routinely used for the synthetic production of snow. These bacteria have proteins in their outer membrane that are able to nucleate ice at temperatures as high as –1 °C. This presentation will discuss our quest to elucidate the mechanisms by which bacterial proteins and other potent ice nucleants promote water crystallization, what makes them so outstanding, and whether we can design materials that outperform them.
The research was funded by the National Science Foundation, the Air Force Office of Scientific Research and the Yen Fellowship from the Institute for Biophysics Dynamics at the University of Chicago.
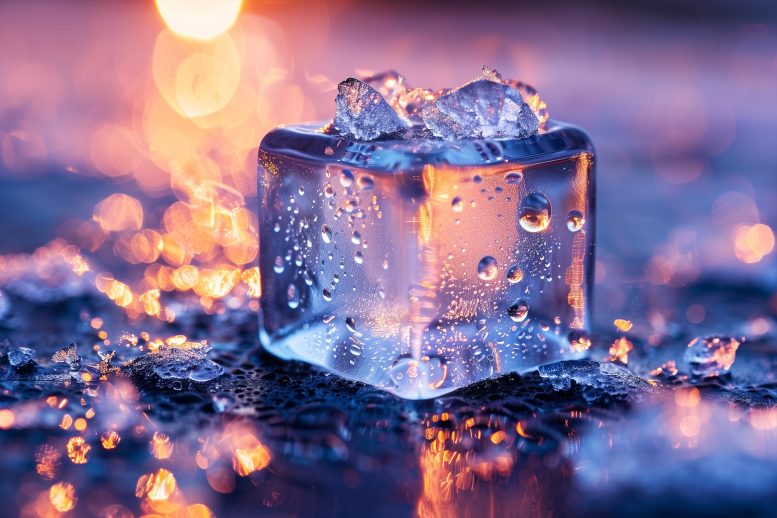
A team of researchers has developed a theoretical model explaining how specific structural details on surfaces influence water’s freezing point, paving the way for advancements in materials design for ice formation and enhanced weather prediction. Credit: SciTechDaily.com
Research unveils a mathematical model for ice nucleation, showing how surface angles affect water’s freezing point, with applications in snowmaking and cloud seeding.
From abstract-looking cloud formations to roars of snow machines on ski slopes, the transformation of liquid water into solid ice touches many facets of life. Water’s freezing point is generally accepted to be 32 degrees Fahrenheit. But that is due to ice nucleation — impurities in everyday water raise its freezing point to this temperature. Now, researchers unveil a theoretical model that shows how specific structural details on surfaces can influence water’s freezing point.
Research Findings and Their Implications
The researchers presented their results at the spring meeting of the American Chemical Society (ACS). ACS Spring 2024 is a hybrid meeting being held virtually and in person March 17-21; it features nearly 12,000 presentations on a range of science topics.
“Ice nucleation is one of the most common phenomena in the atmosphere,” says Valeria Molinero, a professor of physical and materials chemistry. “In the 1950s and 1960s, there was a surge of interest in ice nucleation to control weather through cloud seeding and for other military goals. Some studies addressed how small shapes promote ice nucleation, but the theory was undeveloped, and no one has done anything quantitative.”
Freezing water might seem simple, but not to Yuqing Qiu and Valeria Molinero. By exploring the relationship between surface chemistry and geometry, the process of creating ice or snow could be made more energy efficient, helping create clouds or add snow to mountains. Yuqing will present the research on Wednesday, March 20 at ACS Spring 2024 in New Orleans.
When temperatures drop, the molecules in liquid water, which normally speed around and zip past one another, lose energy and slow down. Once they lose enough energy, they grind to a halt, orient themselves to avoid repulsions and maximize attractions, and vibrate in place, forming the crystalline network of water molecules we call ice. When liquid water is completely pure, ice may not form until the temperature gets down to a frigid –51 degrees Fahrenheit; this is called supercooling. But when even the tiniest impurities — soot, bacteria, or even particular proteins — are present in water, ice crystals can form more easily on the surfaces, resulting in ice formation at temperatures warmer than –51 degrees Fahrenheit.
Advancements in Ice Nucleation Study
Decades of research have revealed trends in how the shapes and structures of different surfaces affect water’s freezing point. In an earlier study on ice-nucleating proteins within bacteria, Molinero and her team found that the distances between the groups of proteins could impact the temperature at which ice formed. “There were distances that were very favorable for ice formation, and distances that were completely opposite,” says Molinero.
Similar trends had been observed for other surfaces, but no mathematical explanation had been found. “People before already had a sense of ‘oh maybe a surface will inhibit or promote ice nucleation,’ but no way to explain or predict what they observed experimentally,” says Yuqing Qiu, a postdoc, who is presenting the work at the meeting. Both Qiu and Molinero carried out this research at the University of Utah, though Qiu now works at the University of Chicago.
To address this gap, Molinero, Qiu, and team gathered hundreds of previously reported measurements on how the angles between microscopic bumps on a surface affected water’s freezing temperature. They then tested theoretical models against the data. They used the models to consider factors that would encourage ice crystal formation, such as how strongly water binds to the surfaces and angles between structural features.
In the end, they identified a mathematical expression that shows that certain angles between surface features makes it easier for water molecules to gather and crystallize at relatively warmer temperatures. They say their model can help design materials with surfaces that would make ice form more efficiently with minimal energy input. Examples include snow or ice makers, or surfaces that are suitable for cloud seeding, which is used by several Western states to increase rainfall. It could also help better explain how tiny mineral particles in the atmosphere help make clouds through ice nucleation, potentially making weather models more effective.
Future Directions in Ice Nucleation Research
The researchers plan to use this model to return to their studies of ice-nucleating proteins in bacteria. More than 200 proteins are believed to be ice-nucleating proteins, but their structures are not all known. The researchers hope to study proteins with structures that have been solved with AI tools, and then they will model how aggregates of those proteins affect ice formation.
Title
The most potent snow makers
Abstract
Several organisms have evolved proteins that control the formation of ice. Ice nucleating bacteria are the most potent ice-nucleating agents in the biosphere and the atmosphere, contributing to cloud glaciation and precipitation, and routinely used for the synthetic production of snow. These bacteria have proteins in their outer membrane that are able to nucleate ice at temperatures as high as –1 °C. This presentation will discuss our quest to elucidate the mechanisms by which bacterial proteins and other potent ice nucleants promote water crystallization, what makes them so outstanding, and whether we can design materials that outperform them.
The research was funded by the National Science Foundation, the Air Force Office of Scientific Research and the Yen Fellowship from the Institute for Biophysics Dynamics at the University of Chicago.